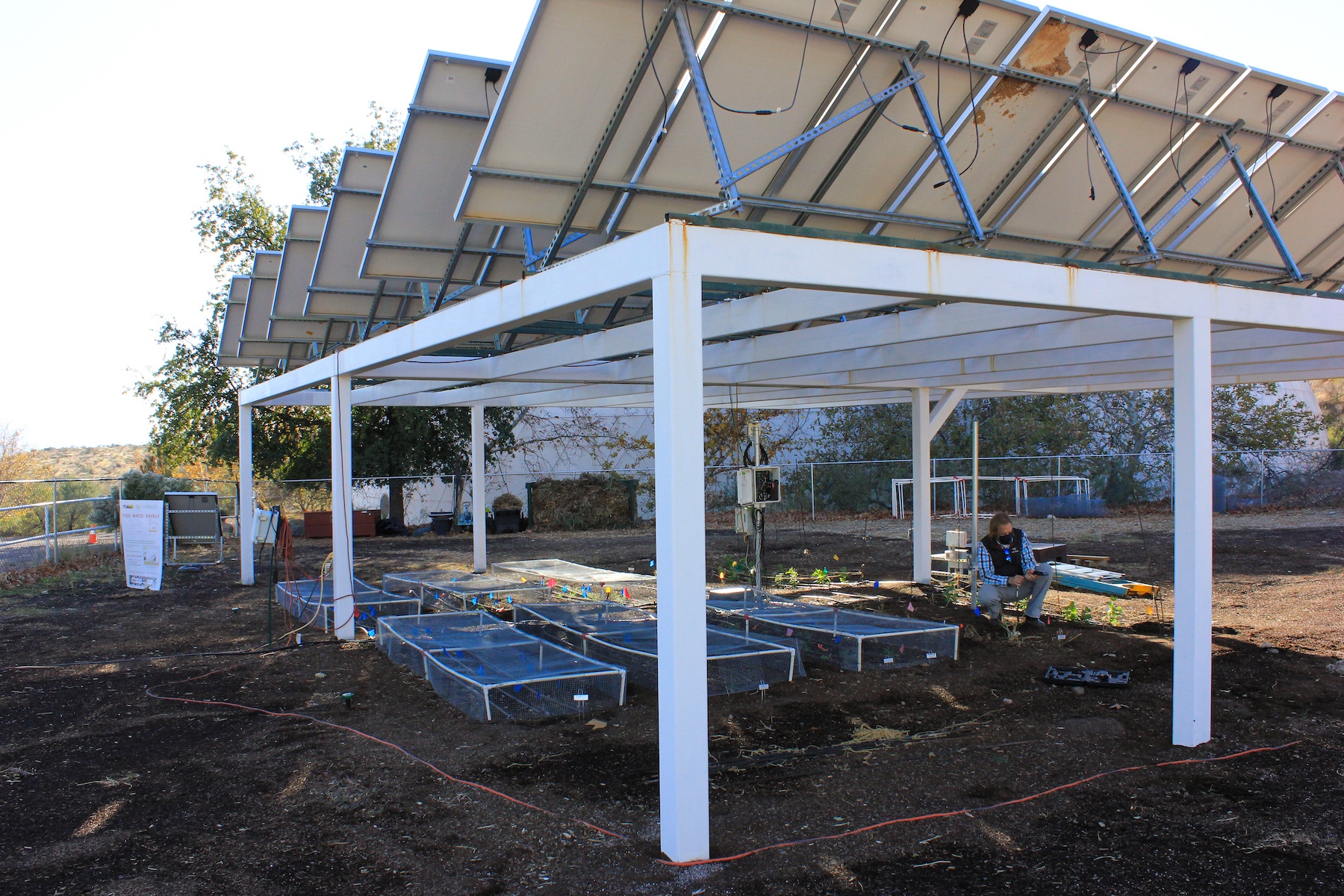
Chris Malloy
Agrivoltaics—putting solar panels on farmland—lead to astonishing productivity gains and improved energy efficiency. Except when they don’t.
On the outskirts of the famed research facility Biosphere 2, away from the futuristic glass pyramid and tiered trapezoids alive with self-contained habitats, University of Arizona biogeographer Greg Barron-Gafford oversees a small outdoor garden. It’s a darker, cooler plot than most, thanks to its distinctive feature: solar panels. Mounted on 9-foot-tall beams, so lofty a tractor could pass below them, the solar panels slant against the blue sky of the high desert, throwing shade on the short rows of basil and onions beneath.
Barron-Gafford has been testing agrivoltaics—a term for land that combines agriculture and solar farming—for 8 years. He started with a single solar panel at Biosphere 2, in Oracle, Arizona, a site the University of Arizona has owned since 2011. More recently, his project has expanded to sites in nearby Tucson and even a large plot overseen jointly by the National Renewable Energy Laboratory (NREL) in Longmont, Colorado.
“Making renewable energy from solar isn’t that complicated anymore,” said Barron-Gafford, crouching by a shadow-thatched row of carrots to check soil moisture, to be compared to a nearby control garden without an overhead solar system. “The price has really come down. The question is, where do you put it?”
“Making renewable energy from solar isn’t that complicated anymore.”
It’s a good question. In order to address climate change, President Joe Biden campaigned on a plan for the U.S. to move to clean electricity by 2035. In 2019, 11 percent of U.S. energy came from renewable sources. Just some 2 percent was solar. To reach the 2035 goal, solar will very likely need a much bigger slice of our energy portfolio.
[Subscribe to our 2x-weekly newsletter and never miss a story.]
That, though, will require expansion over land. Like windmills, solar panels need space, creating what has been dubbed “energy sprawl.” Even as a fraction of the U.S. energy portfolio, solar power has already led to land-use conflicts, with proponents of solar starting to spar with farmers over land.
Agrivoltaics help to solve that spatial dilemma. They allow a given area to harvest the sun not only once, but twice — as fuel for crops and as a source of renewable energy. But space-saving isn’t all that has sparked the interest of researchers and advocates across the world, from the U.S. to Western Europe and Japan. Another kind of symbiosis can occur, with surprising benefits we are only just beginning to understand—an untapped synergy that may have the potential to transform the way we produce both food and energy.
—
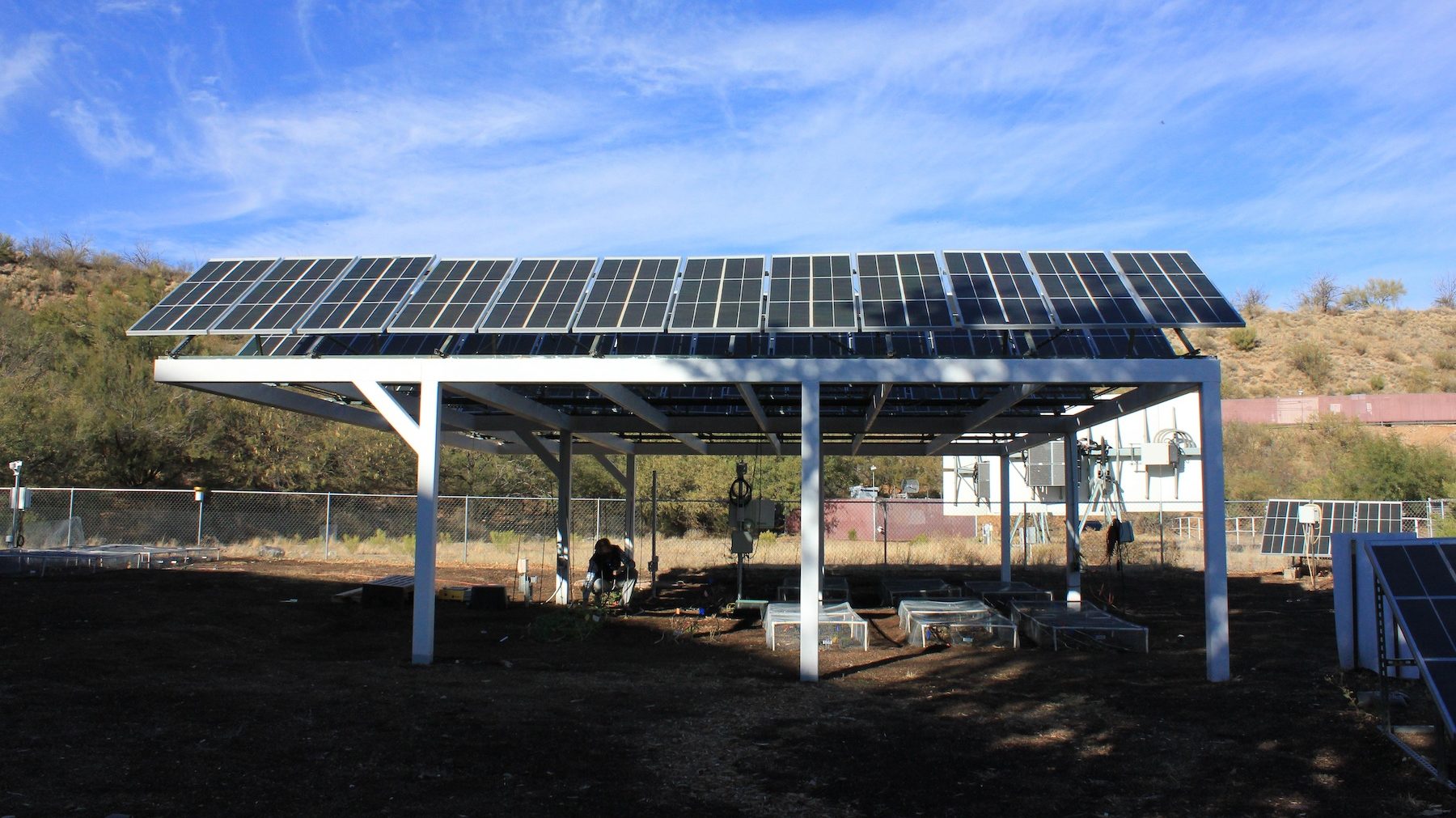
Above, an experimental garden plot at the University of Arizona’s Biosphere 2 complex. In agrivoltaic systems, the partial shade created by solar panels can benefit some crops in some climates.
Chris Malloy
For Barron-Gafford, the idea emerged organically from a challenge in his work. In 2012, he was looking for ways to quantify the temperature of large solar arrays, trying to determine how they might contribute to an urban heat island effect, or the phenomenon of cities being warmer than the surrounding countryside. He noticed that heat collected beneath the solar array, making the panels less efficient. Maybe, he thought, growing plants under the panels would cool them. Plants, after all, “exhale” water through a process called transpiration, losing water through their pores as they intake carbon dioxide for photosynthesis.
Indeed, Barron-Gafford’s agrivoltaic garden proved to produce a cooler microclimate under panels than conventional solar farms, something other studies have echoed with findings from their own plots. Intrigued, he went on to research the phenomenon in more detail. In his 2019 study of jalapenos, cherry tomatoes, and chiltepines (a desert chile native to the U.S.), Barron-Gafford found that agrivoltaic panels produced 3 percent more energy during the May-through-June growing season and 1 percent more overall.
But the benefits weren’t only on the energy-efficiency side. His simple system—just solar panels lofted above plants—also helped the crops, which needed far less water to grow. This was due to cool shade under the solar panels. “If you spilled your water bottle in the shade versus out in the sun, where’s it going to stay wet longer? In the shade,” he said. “So we’re just using these oh duh principles to try to make a more sustainable food system.”
But the shade also conferred another significant benefit, one that may prove tantalizing for farmers focused on yield: It caused Barron-Gafford’s plants to grow differently.
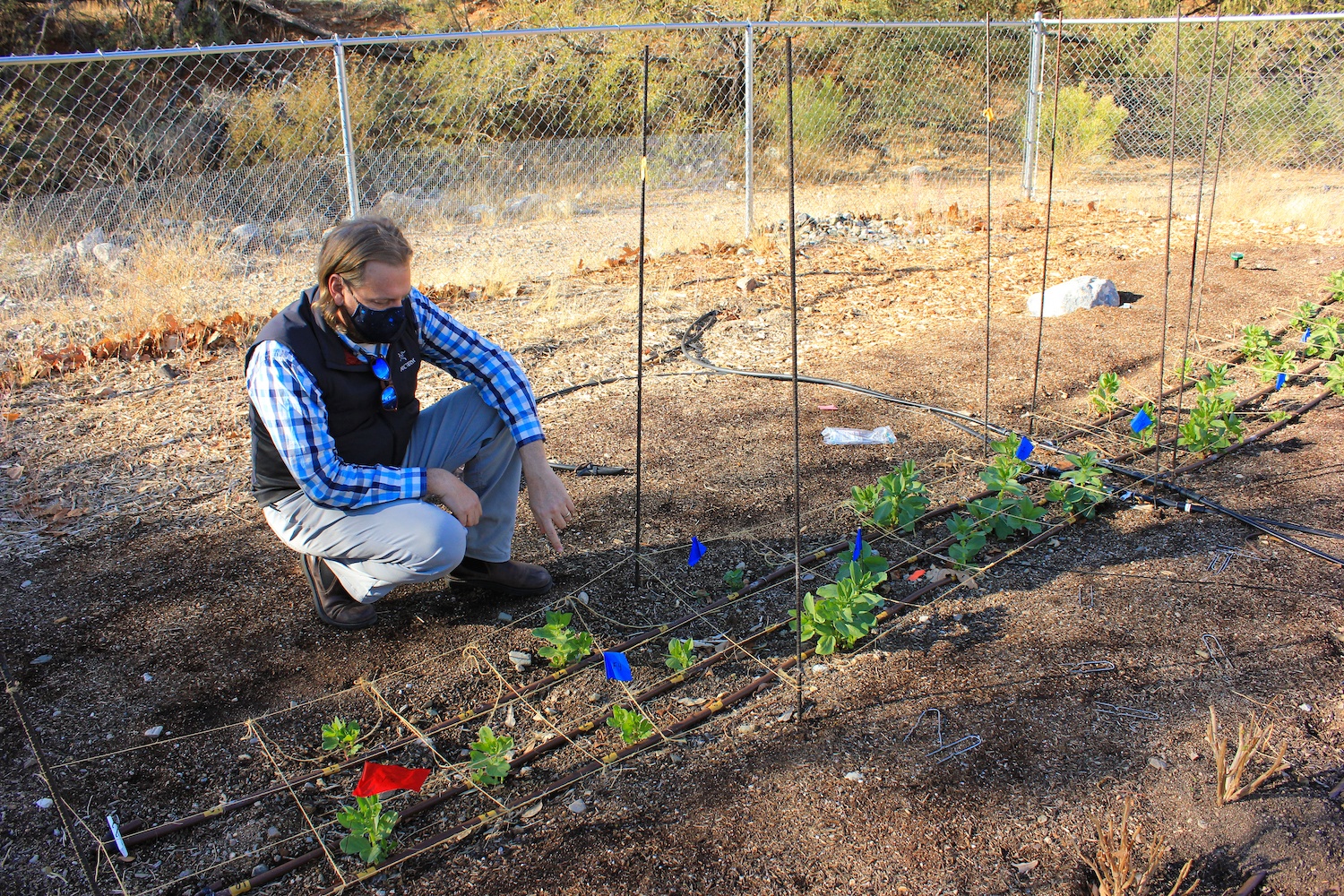
University of Arizona scientist Greg Barron-Gafford observes the progress of a control garden.
Chris Malloy
Some plants thrive in partial sun, especially in semiarid regions where farms may be growing non-native crops unadapted to water scarcity, like the Southwest. That makes solar panels an ideal growing partner. The shade they provide keeps moisture in the ground longer, which keeps plants from stressing out, Barron-Gafford explained; instead of burning through their energy reserves early, crops can keep photosynthesizing at a more even, measured rate throughout the day.
In some species, that leads to markedly increased production, Barron-Gafford found: The presence of plants ups solar energy production, and solar panels returned the favor for two of the three plants he studied. Tomato production doubled, with 65 percent more water efficiency. Chiltepin production tripled. Jalapeno production was static, yet with water efficiency 157 percent greater — a win in a region embroiled in historic drought.
While those numbers represent astonishing productivity gains, similar results may not be possible in every region or with every crop, said Jordan Macknick, lead energy-water-land analyst for NREL. Macknick has studied agrivoltaics with NREL since 2010, working to understand “what grows under solar panels where and why.” Today, he analyzes data from 25 sites, including the plot at Biosphere 2, together forming “a network of projects that are designed to explore the tradeoff between solar energy development and agriculture.”
It’s clear that what works in Arizona might not work in the Pacific Northwest, and what works in New England might not work in the South. “One of the challenges is many things in agrivoltaics are very site specific,” Macknick said. “You have to try very hard to be able to extrapolate results from one region to another region.” His research partners take a consistent approach to enable comparison.
“You have to try very hard to be able to extrapolate results from one region to another region.”
Agrivoltaics projects across the world already demonstrate some of this inherent variability. In Minnesota, solar panels shading the cattle of a rotational grazing pasture have successfully lowered animal heat stress while powering a milking parlor. In Belgium, an agrivoltaic potato field showed cooler microclimates below panels and less evaporation, as well as larger leaves with “adapted light harvesting capability.” But in other locations, the results are more mixed. In Massachusetts, numerous cranberry bog farmers have adopted agrivoltaics, hoping that solar energy will provide an additional source of revenue and offset plummeting prices—though some worry that the crop won’t respond as well to shade, the Associate Press reports. In Italy, spinach yields decreased in one study. Still, its researchers observed more efficient photosynthesis and greater protein production. They calculated that the financial value of the agrivoltaic spinach remained greater, given the solar energy production.
Generally, research suggests that agrivoltaics might benefit, at the very least, a small fraction of global farmland. But agrivoltaics won’t need to work everywhere to become a transformative idea. Writing in the journal Scientific Reports, scientists from Oregon State University found that if a mere 1 percent of global cropland gained solar panels, “global energy demand would be offset by solar production.”
Think about that for a second: turning just 1 percent of cropland agrivoltaic—a method that can also increase harvests in many environments—could more than satisfy the world’s energy demand, generating BTUs by the hundred quadrillion.
—
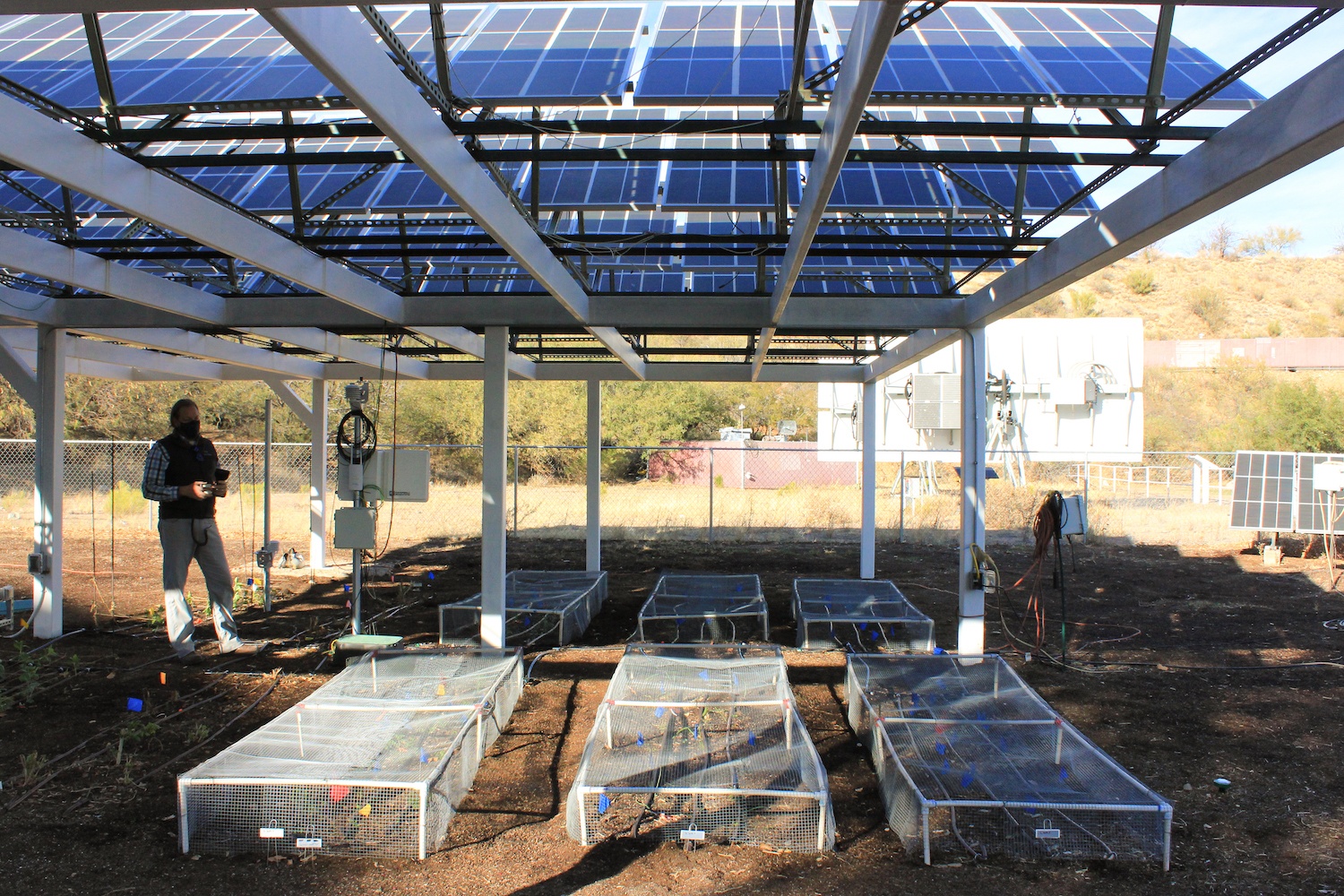
Adopted at scale, agrivoltaic farms might help the U.S. achieve ambitious clean electricity plans. Putting solar panels on only 1 percent of the world’s cropland could more than satisfy global energy demand.
Chris Malloy
Though sensible in theory and simple in imagination, turning visions of solar-paneled farms into reality comes with its own challenges. Agrivoltaic systems are no panacea: they can introduce new uncertainties, exacerbate old complexities, and many questions remain.
First, there is the question of farming logistics. Crops that require aerial spraying, like cotton, likely won’t thrive in agrivoltaic systems. There have been concerns about how rain might run from panels and cause erosion, though these concerns were unfounded in both a German study and in Barron-Gafford’s work. Also, low panels could obstruct farm vehicles. Barron-Gafford and other experimenters tend to keep panels 6-to-9 feet off the ground — too low for some large combines, but high enough for many tractors. These vehicles can raise dust onto panels, cutting efficiency. A study notes that self-cleaning panels would help, and so would tweaking irrigation to clear panels before dripping to crops, “thus facilitating effective water usage.”
Second, crop constraints loom. Some plants dislike partial sun. Corn, for instance, has shown weaker stalk growth and lower photosynthesis rates in shade. Scientists like Barron-Gafford are still determining which crops do best under panels.
More broadly, agrivoltaics introduce new variables to the business of farming, which is already chock full of them. One undesirable factor: lightning can strike solar panels. A study anticipates these will happen about once every 30 years and can be averted via lightning rods.
“If I visit my sheep at lunchtime, under the panels they’re asleep, they’re chewing their cud, they are not stressed. And they drink less water than they would if I had them home here on the farm.”
Other challenges are more vexing. In a survey conducted by Michigan Technological University, farmers expressed concerns over not knowing how crop or livestock markets might look in the years ahead. A farmer who makes an investment in agrivoltaics must look 10, 20, 30 years into the future to project revenue, and calculating profit margins is still a speculative exercise. Additionally, some farmers surveyed wondered how the concrete and beams might change the land over time, with an influx of large, permanent structures potentially causing issues for crops or for livestock.
Perhaps most significantly, the upfront costs of agrivoltaics are giant. They will have to be defrayed through some combination of policy, tax incentives, favorable loans, and/or integration into electrical grids, where farmers can profit from selling the sunlight they farm. In the end, the balance of upfront capital costs for installation, solar energy sales, and agriculture sales must exceed the sales of a traditional farm.
These novel challenges have led to some unusual partnerships and approaches. Solar grazing, for instance, typically means bringing in livestock from outside farms and ranches to help maintain a solar site.
“With the solar… the animals have shade in abundance,” said Lexie Hain, executive director of the American Solar Grazing Association, who practices solar grazing with sheep in upstate New York. “If I visit my sheep at lunchtime, under the panels they’re asleep, they’re chewing their cud, they are not stressed. And they drink less water than they would if I had them home here on the farm.”
Like many solar grazers, Hahn transports her sheep to solar farms to graze. The owners need vegetation trimmed so it doesn’t block or damage panels—so, for a price and feed for their animals, solar grazers step in. “I can do it for about half of what the landscapers do,” saidRichard Cocke, a shepherd who brings his sheep to two solar sites in southern Arizona, having stopped visiting a third due to coyote troubles. “I don’t cause breakage to the panels. And it’s green.”
Pollinator-friendly sites are another sub-category. According to Yale research, this approach—which entails growing beneficial non-food plants like perennial wildflowers and native grasses under solar cells—can increase groundwater recharge, curb soil erosion, and up solar efficiency by creating a cooler microclimate for panels. It can also bolster crop yields on nearby farms of crops requiring pollination. But large-scale adoption, the study authors write, will “warrant policy intervention.” With no crop to sell, pollinator-focused solar farmers would likely need lawmakers and business leaders to recognize the ecosystem services they provide, ultimately developing systems of financial incentives to make the extra trouble worth it.
“Maybe after some years, after our researchers are done studying here… we’ll have a sense of what we can do with the land underneath solar panels.”
The need for supportive policy is echoed down the chain of people involved in agrivoltaics, including by Byron Kominek, the owner of Jack’s Solar Garden, a site with which Macknick and Barron-Gafford work. This Longmont, Colorado agrivoltaic plot is the largest in America.
Completed in late 2020, the 3,200-panel solar system at Jack’s Solar Garden spans five acres and can power more than 300 homes. Previously, the farm produced hay. Now, Kominek will grow vegetables and whatever researchers want to test, largely for data collection. “Maybe after some years, after our researchers are done studying here… we’ll have a sense of what we can do with the land underneath the solar panels and [can] start growing,” Kominek said.
To start, Jack’s makes money by selling energy. It has subscribers that, through a local energy company, buy power from its solar grid almost like you would produce from a CSA. The biggest subscriber is a local cannabis company. These cash infusions have helped with the “big expense” of installation. “We were able to cover it because we basically asked our subscribers to pay us upfront for X number of years,” Kominek said. “This helps us have the upfront capital that we can payoff our engineers who helped build the system.”
“In the end, these things only pan out if the finance is there. They don’t work on hopes and dreams.”
Kominek hopes that legislators will look at Barron-Gafford’s projects and other developments, like the budding scene in Massachusetts, the only state with a financial incentive for agrivoltaics (6 cents per kilowatt-hour). He hopes the practice can be fostered via rulemaking.
“In the end, these things only pan out if the finance is there,” he said. “They don’t work on hopes and dreams.”
—
At sites like the agrivoltaic garden on Biosphere 2, a complex built to test how people might live on other planets, we are still learning clues for living on this one.
Barron-Gafford’s young winter crop includes six plants, like chickpeas and fava beans, which will also appear in a sister garden run by The Arava Institute for Environmental Studies in the Arava Desert of Jordan and Israel, home to a climate similar to the desert of southern Arizona. Increasingly, agrivoltaics researchers are collaborating to suss out the nuances of the technology, which—though expensive, not for every climate or crop, and arguably an aesthetic blight on the land—packs layers of potential.
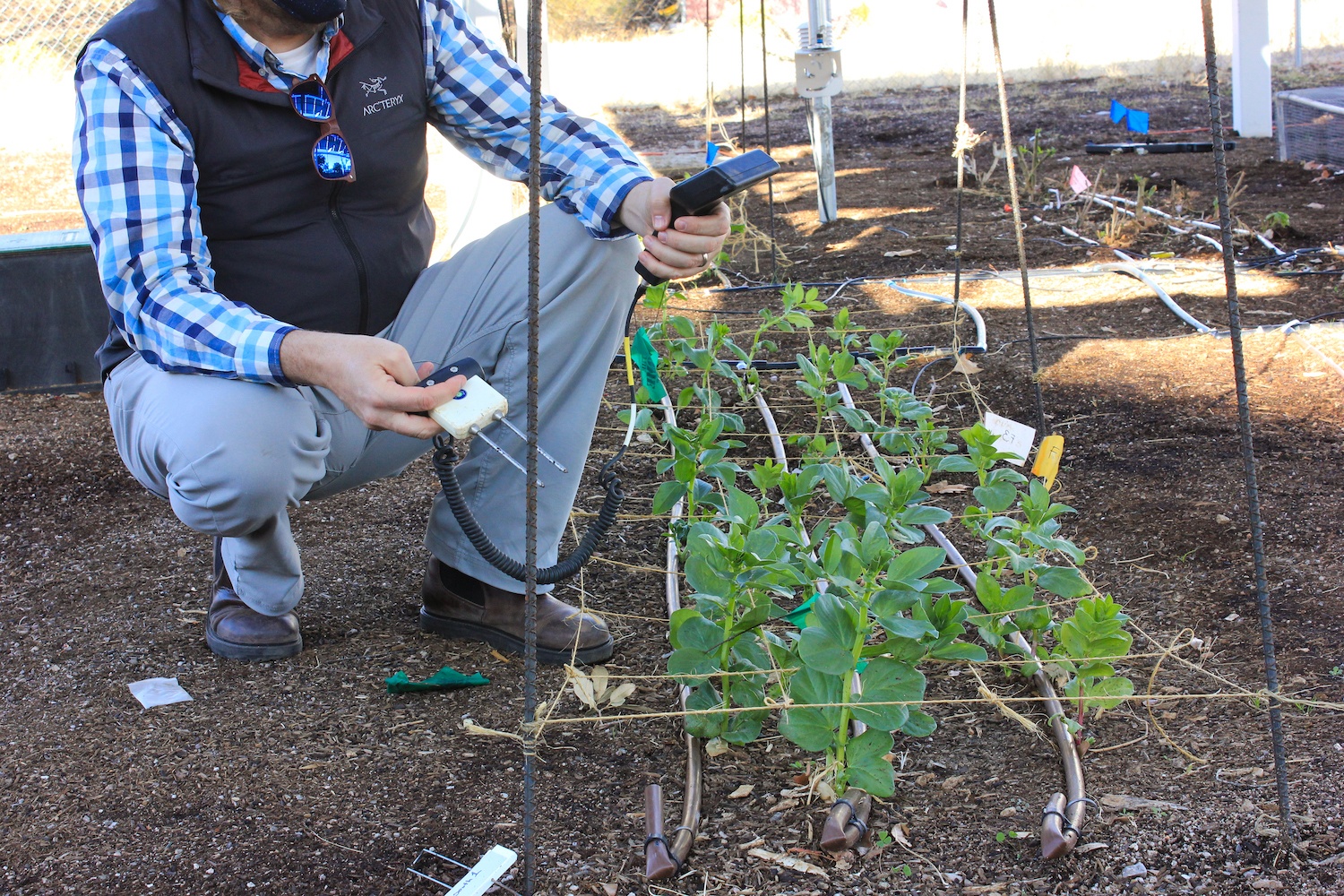
At the Biosphere 2 plot in Oracle, Arizona, Barron-Gafford squats by agrivoltaic fava beans and collects data with a soil water content probe.
Chris Malloy
Standing with his boots in the dirt of the American county with the second-most climate risk, Barron-Gafford ponders the future ahead. “What happens in another 50 years when we’re under increased pressure because of dropping reservoirs?” he asks. “What about 50 years when our temperatures are beyond what plants can handle?”
For the U.S. to meet its electricity goals and to avert greater climate chaos, renewable energy will likely have to grow. So the fact that the world could meet energy demand by adding solar panels to 1 percent of cropland suggests agrivoltaics are a condundrum worth puzzling over. With the right policies, incentives, and farmer support, the technology might be a rare win-win. It might even be low-hanging fruit.